The Role of RNA in Protein Synthesis
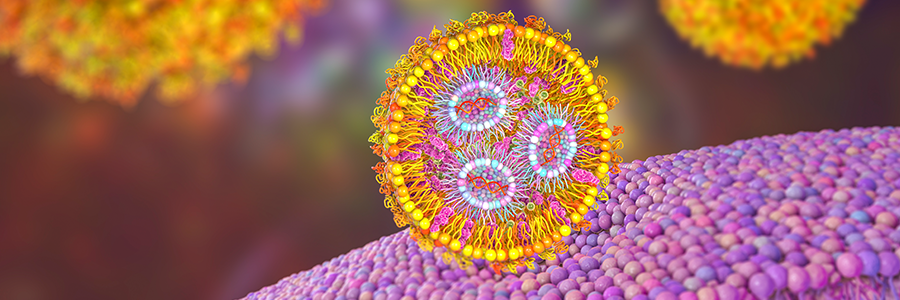
Ribonucleic acid (RNA) plays a crucial role in protein synthesis. Protein synthesis occurs in two main stages: transcription and translation.
During transcription, the DNA sequence of a gene is used as a template to synthesize a messenger RNA (mRNA) molecule. This mRNA molecule carries the genetic information from the DNA to the ribosomes.
Once the mRNA molecule reaches the ribosome, the translation process commences. It involves using transfer RNA (tRNA) molecules to bring amino acids to the ribosome, which are linked to form a polypeptide chain. The sequence of nucleotides in the mRNA molecule determines the sequence of amino acids in the polypeptide chain, which helps analyze the structure and function of the protein.
RNA plays an important role in both transcription and translation. During transcription, RNA polymerase enzymes catalyze the synthesis of mRNA molecules from DNA templates. These enzymes ensure that the mRNA molecule is complementary to the DNA template, allowing for the accurate transfer of genetic information.
During translation, tRNA molecules serve as adaptors, binding to specific amino acids and recognizing the codons in the mRNA sequence corresponding to those amino acids. Subsequently, rRNA is an important structural component of the ribosome. It exerts the necessary physical and mechanical force to facilitate the processing and translation of mRNA, leading to protein synthesis. These are discussed below.
What is the function of ribosomes?
The main function of ribosomes is to read the genetic instructions encoded in messenger RNA and use this information to build a specific sequence of amino acids into a protein. Ribosomes usually accomplish this by catalyzing the formation of peptide bonds between adjacent amino acids to elongate the growing protein chain further.
Ribosomes consist of two subunits, each composed of ribosomal RNA (rRNA) and various proteins. The smaller subunit binds to the mRNA, while the larger subunit catalyzes the formation of peptides between the amino acids.
Ribosomes can be found in two main locations within cells: free in the cytoplasm or attached to the endoplasmic reticulum (ER). Free ribosomes synthesize proteins to use within the cytoplasm. At the same time, those attached to the ER are synthesizing proteins to transport them to other parts of the cell or secrete them outside the cell.
The three types of RNA in protein synthesis
As discussed above, protein synthesis occurs in cells and involves synthesizing proteins from amino acids. The three main types of RNA involved in protein synthesis are messenger RNA, transfer RNA and ribosomal RNA. These are discussed in detail below.
Messenger RNA
Messenger RNA is a single-stranded RNA molecule that carries genetic information from DNA in a cell’s nucleus to the ribosomes, serving as a template for protein synthesis. The mRNA is synthesized through transcription. It carries the genetic code for a specific protein, which is determined by the sequence of nucleotides in the mRNA.
The mRNA serves as a template for the protein synthesis. The ribosomes read the sequence of nucleotides in the mRNA and use this information to assemble a specific sequence of amino acids, eventually forming a protein.
Moreover, the mRNA translation is subject to regulation that results in dynamic modulation of protein expression levels. This regulation specifically occurs through specific RNA sequences and structures present in the mRNA transcript’s untranslated regions. These features are usually recognized by regulatory proteins or micro RNAs (miRNAs).
Transfer RNA
Transfer RNA is a small molecule carrying amino acids to the ribosome during protein synthesis. Each tRNA molecule has a specific sequence of three nucleotides (called the anticodon) complementary to a specific codon in the mRNA. The tRNA binds to the amino acid corresponding to its anticodon, where it is added to the growing protein chain.
The tRNA delivers the correct amino acid to the ribosome based on the information the mRNA provides. The tRNA also ensures that the correct amino acid is added to the protein chain, as the anticodon must match the codon in the mRNA.
Ribosomal RNA
Ribosomal RNA is an RNA type that is a structural component of ribosomes, the cellular organelles responsible for protein synthesis. The rRNA is transcribed from DNA in the cell’s nucleolus and combines with proteins to form ribosomal subunits. The ribosomal subunits then assemble on the mRNA and catalyze the formation of peptide bonds between adjacent amino acids.
The rRNA, being a structural component of ribosomes, is essential for protein synthesis. The rRNA also plays a role in catalyzing the formation of peptide bonds between adjacent amino acids during protein synthesis.
What is the structure of RNA?
The structure of RNA comprises nucleotides consisting of three components: a nitrogenous base, a five-carbon sugar and a phosphate group. The four nitrogenous bases found in RNA are adenine (A), cytosine (C), guanine (G) and uracil (U).
Adenine and guanine are purines, while cytosine and uracil are pyrimidines. The sugar component in RNA is called ribose, which differs from deoxyribose found in DNA due to an additional hydroxyl (-OH) group attached to the 2' carbon atom.
The RNA’s primary structure is the sequence of nucleotides linked by phosphodiester bonds between the 3' hydroxyl group of one nucleotide and the 5' phosphate group of the next. This sequence determines the genetic information that is carried by the RNA molecule.
The RNA’s secondary structure is how the RNA molecule folds into a specific shape, determined by the sequence of nucleotides. RNA molecules can form secondary structures, such as hairpin loops, bulges, internal loops, and multi-branched structures.
The secondary structure is stabilized by hydrogen bonds between complementary base pairs. Adenine always pairs with uracil, and guanine always pairs with cytosine.
The RNA’s tertiary structure is the overall three-dimensional structure of the RNA molecule, determined by the interactions between different parts. These interactions can include hydrogen bonds, electrostatic interactions, hydrophobic interactions and van der Waals interactions. Tertiary structures can be very complex, including long-range interactions that bring distant molecule parts together.
How Is RNA Made?
RNA is synthesized through transcription, which involves copying genetic information from DNA into RNA. The genetic information in DNA is encoded in its nucleotide sequence, which is transcribed into RNA in a complementary sequence.
The transcription process begins when RNA polymerase binds to a specific region of DNA called the promoter. This region contains the information for the RNA polymerase to start transcription.
RNA polymerase then moves along the DNA strand, unwinding the double helix and reading the nucleotide sequence. As RNA polymerase moves along the DNA strand, it adds complementary RNA nucleotides to the growing RNA strand in a 5' to 3' direction.
In prokaryotes, transcription occurs in the cytoplasm, and the RNA is immediately available for translation into proteins. While in eukaryotes, transcription occurs in the nucleus, and the RNA is processed before it is transported to the cytoplasm for translation.
The initial RNA transcript produced during transcription is called pre-mRNA. Pre-mRNA contains both coding and non-coding regions and must be processed before being translated into protein. RNA processing includes three steps: capping, splicing and polyadenylation.
Capping involves the addition of a 5' cap to the pre-mRNA, which prevents RNA degradation and helps it to be recognized by the translation machinery. Splicing involves the removal of non-coding regions called introns and the joining of coding regions called exons.
This process produces a mature mRNA molecule that can be translated into protein. Finally, polyadenylation involves the addition of a poly(A) tail to the 3' end of the mRNA, further stabilizing the RNA molecule and promoting its translation.
What does RNA do in protein synthesis?
RNA in protein synthesis serves as an intermediary between DNA and proteins. mRNA, tRNA and rRNA are involved in this synthesis process. mRNA carries the genetic information encoded in DNA from the nucleus to the ribosomes in the cytoplasm.
The ribosomes use this information to synthesize specific proteins. mRNA is transcribed from DNA in the nucleus and undergoes several modifications before being transported to the cytoplasm.
tRNA carries amino acids to the ribosome during translation. Each tRNA molecule is specific to one amino acid and contains a three-nucleotide sequence, or anticodon, that matches the codon on the mRNA. The tRNA molecule delivers the amino acid to the ribosomes, adding it to the growing protein chain.
Finally, rRNA plays a structural role in facilitating protein synthesis. The ribosome comprises two subunits, each containing rRNA and many different proteins. The rRNA molecules in the ribosome interact with the mRNA and tRNA molecules to facilitate the protein assembly.
Complications within RNA
RNA plays a crucial role in the process of protein synthesis. However, this process can be complicated due to various RNA-related factors, leading to errors during protein synthesis, resulting in non-functional or aberrant proteins. These complications are listed below.
RNA Instability: RNA is inherently unstable and can degrade easily, causing errors during protein synthesis or even complete failure.
RNA Processing: RNA undergoes several post-transcriptional modifications, such as splicing, editing and polyadenylation, affecting its stability and function. Errors in RNA processing can result in aberrant transcripts that do not function properly in protein synthesis.
RNA Folding: RNA has a complex three-dimensional structure that is essential for its function in protein synthesis. However, improper folding of RNA molecules can interfere with their ability to interact with ribosomes and other translation machinery components.
RNA Modification: RNA molecules can undergo various modifications, such as methylation, affecting their stability and function. Some modifications can even regulate gene expression by altering RNA processing or translation.
RNA Editing: In this process, the RNA’s nucleotide sequence is altered after transcription. As a result, errors in RNA editing can result in non-functional or aberrant proteins being synthesized.
RNA Splicing: In this process, the introns are removed from pre-mRNA, and exons are spliced together to form mature mRNA. Errors in RNA splicing can result in the inclusion of introns or the exclusion of exons, leading to non-functional or truncated proteins.
RNA Mutations
RNA mutations are changes in the nucleotide sequence of RNA molecules affecting their stability and function. RNA mutations can occur spontaneously during DNA replication or transcription, or they can be induced by mutagens such as chemicals, radiation or viruses. RNA mutations can be silent, missense or nonsense, depending on their effect on the synthesized protein’s amino acid sequence.
Silent mutations do not affect the protein sequence, while missense mutations substitute one amino acid for another. Nonsense mutations form a premature stop codon, leading to the synthesis of truncated or non-functional proteins.
RNA mutations are quite common, with the rate of spontaneous mutations varying depending on the type of RNA and the organism. For example, the rate of spontaneous mutations in human mitochondrial RNA is estimated to be 10-20 times higher than in nuclear RNA.
RNA mutations can have various risks depending on location, type and frequency. Some RNA mutations can cause genetic diseases or disorders, such as cystic fibrosis, sickle cell anemia and Huntington’s disease.
Other RNA mutations may have little or no effect on protein function. At the same time, some may even confer a survival advantage, as in the case of sickle cell anemia, which can confer resistance to malaria.
mRNA has grown its popularity and importance in many therapeutic areas due to its wide range of applications, including vaccine, gene editing and many more. The manufacturing process and CMC characteristics are relatively more straightforward than that of the larger molecules, such as CAR-T or adeno-associated viral (AAV) vector.
If you’re interested in learning more about the role of mRNA in protein synthesis, Avantor Sciences offers various resources on life science to explore, from scientific articles to educational videos. Whether you’re a student or researcher, we have something for you. View our resources and discover the wonders of mRNA and protein synthesis. Visit Avantor Sciences to learn more!