Monoclonal Antibodies: Understanding Their Production and Clinical Applications
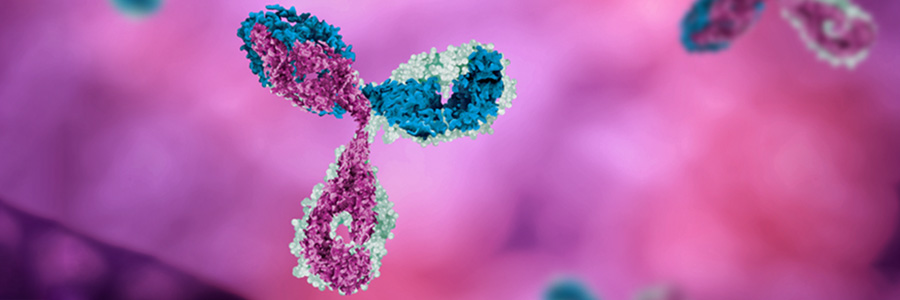
Monoclonal antibodies, mAbs, have transformed the field of medicine, thanks to their remarkable specificity and effectiveness in treating various diseases. This article will delve into monoclonal antibody production and clinical applications to provide a thorough understanding of their role in healthcare.
What are monoclonal antibodies?
Monoclonal antibodies are laboratory-produced antibodies designed to mimic the natural antibodies produced by the immune system. Their purpose is to target specific molecules or cells in the body, aiding in diagnostics, therapy, or research. These are created using hybridoma technology, where a specific B cell is isolated and fused with a myeloma (not cancer) cell to form a hybridoma cell line capable of producing large quantities of identical antibodies. A hybridoma is a fused cell line formed by combining a specific B cell and a cancer cell, and is used for production of monoclonal antibodies. The cell can produce large quantities of identical monoclonal antibodies.
Development of monoclonal antibodies
The history of monoclonal antibodies dates back to the 1970s, highlighting several scientific breakthroughs toward their development. In 1975, Georges Köhler and César Milstein introduced the hybridoma technology, laying the foundation for monoclonal antibody production. They received the Nobel Prize in Medicine in 1984 for such a groundbreaking work of revolutionizing mAb production. The hybridoma technology involves antibody-producing B cells with immortalized myeloma cells to generate hybrid cells that can produce a specific antibody.
The monoclonal antibodies were first successfully produced in 1975 when Köhler and Milstein generated hybridoma cell lines that secreted antibodies against the immunoglobulin of a mouse. This achievement opened the doors to a new antibody research and development period.
Monoclonal antibodies, originally used for immune system research and molecule identification, have now found applications in diverse fields. However, further research and analysis revealed their potential for therapeutic application.
An early therapeutic application of monoclonal antibodies was the development of the anti-CD3 antibody OKT3 in 1986. OKT3 was used to prevent organ rejection in transplant patients by modulating the immune response. This marked the beginning of the use of monoclonal antibodies in clinical practice.
Over the years, the development of monoclonal antibodies expanded rapidly. Moreover, advances in antibody engineering and modification techniques helped create monoclonal antibodies that were developed and approved for various indications, such as cancer, autoimmune diseases and infectious diseases.
In recent years, monoclonal antibodies have evolved with new approaches, including transgenic animals, in-vitro antibody production and microbial systems, to improve production efficiency and reduce costs. Furthermore, combining monoclonal antibodies with other therapeutic modalities, such as immune checkpoint inhibitors and small molecule inhibitors, has shown promising results and opened up new avenues for treatment. The history of monoclonal antibodies is represented in the flow chart provided below.
Importance of monoclonal antibodies in biopharma
Monoclonal antibodies have become indispensable tools in the field of biopharmaceuticals. These antibodies play a vital role in both biomedical and clinical applications by selectively targeting and binding to specific molecules or cells, for facilitating diagnosis, treatment, and research advancements.. Their unique properties and capabilities such as high specificity and affinity for their target, low toxicity, long half-life, and the ability to engage the immune system have helped revolutionize various areas of biopharma. Some ways in which they have benefitted the biopharma industry are provided below:
● Therapeutic Efficacy
Monoclonal antibodies are a highly effective class of therapeutics. Their specificity makes them ideal for treating various types of diseases. Monoclonal antibody therapeutics have successfully helped treat cancer, autoimmune disorders and more. They can directly neutralize pathogens, modulate immune responses, curb tumor growth and improve the body’s natural defences against diseases.
● Targeted Precision
These antibodies can be engineered to recognize and bind to precise targets specifically. The precision enables selectively targeting disease-causing cells or molecules while reducing the impact on healthy tissues. This targeted approach minimizes side effects and enhances the safety profile of therapeutic interventions.
● Diagnostic Tools
Monoclonal antibodies are important in diagnostic applications as they are used in techniques such as ELISA, western blotting, immunohistochemistry and flow cytometry. These allow the researchers to detect, quantify and visualize specific molecules or cells, enabling accurate disease detection and biomarker identification for personalized medicine.
● Research Tools
These antibodies serve as essential research tools in biomedical research. They help scientists understand disease mechanisms, characterize cellular and molecular processes, and identify some potential drug targets. Moreover, these also help study the protein-protein interactions, cell signaling pathways and immune responses, providing important insights into disease biology.
● Advancements in Antibody Engineering
Antibody engineering techniques have enhanced and expanded the outreach of monoclonal antibodies. Researchers can modify and optimize antibodies to improve their efficacy, stability and half-life. This engineering technique has helped develop antibody-drug conjugates, bispecific antibodies, immune checkpoint inhibitors and more. Moreover, these advancements have broadened the therapeutic options and increased the potential for personalized medicine.
● Drug Development and Commercialization
Monoclonal antibodies have transformed the landscape of drug development and commercialization. They have become a significant class of biopharmaceuticals, with numerous monoclonal antibody-based therapies gaining regulatory approvals. The success of monoclonal antibodies has paved the way for a robust biopharmaceutical industry, contributing to further research and development.
Production of monoclonal antibodies
Production of monoclonal antibodies is a complex and step-by-step process due to factors such as immunization, B cells production, screening, and finally culturing and scaling to produce large quantities of mAbs for various biomedical and clinical applications.
Principles of hybridoma technology
Hybridoma technology involves the fusion of a specific antibody-producing B cell with a myeloma cell (an immortalized cancerous plasma cell). This fusion creates hybrid cells known as hybridomas that can produce large amounts of a single antibody type.
The B cells and myeloma cells are typically isolated from mice or other potential animals to create hybridomas. While B cells are stimulated with the target antigen to trigger antibody production, myeloma cells are selected based on their ability to grow rapidly in culture and their lack of antibody production.
Then, both the cells are fused using various techniques, such as chemical fusion agents or electrical stimulation. After fusion, the resulting hybridoma cells are cultured in a selective medium that supports the growth of hybridomas only.
This medium usually contains a substance that prevents the growth of unfused myeloma cells, allowing only the hybridomas to survive. The hybridomas are then screened to identify those producing the desired monoclonal antibodies.
Steps in producing monoclonal antibodies
Producing monoclonal antibodies using Chinese hamster ovary (CHO) cells involves a series of steps. The production of monoclonal antibodies through CHO cells is a complex and intricate process that requires careful planning, expertise, and quality control measures at every step. The steps in producing monoclonal antibodies can be outlined as follows: A
Antigen Selection: The first step is to identify and select the specific antigen against which the monoclonal antibody will be developed. This antigen could be a protein, peptide, or any other molecule of interest.
Immunization: Animals, typically mice, are immunized with the chosen antigen. This process stimulates the immune system to produce a diverse range of antibodies against the antigen.
Cell Fusion: After a sufficient immune response has been generated, antibody-producing cells (B cells) are extracted from the immunized animal, usually from the spleen or lymph nodes. These cells are fused with myeloma cells, a type of cancerous cell that can divide indefinitely. The resulting fused cells are called hybridomas.
Hybridoma Screening: The hybridoma cells are cultured in a specialized medium that supports their growth. The culture medium also contains a selective agent that allows only the hybridoma cells to survive while inhibiting the growth of unfused myeloma cells and other cells. This process is known as selective medium or HAT (hypoxanthine, aminopterin, and thymidine) selection.
Cloning: The surviving hybridoma cells are then seeded into individual wells of a culture plate through a process called limiting dilution. This step ensures that each well contains only one hybridoma cell, allowing for the isolation of monoclonal populations.
Antibody Production: The selected monoclonal hybridoma cells are further grown in a larger scale to produce a larger quantity of antibodies. This is usually done in bioreactors or large-scale cell culture systems.
Antibody Purification: The culture supernatant or the harvested cells are processed to extract the monoclonal antibodies. This purification involves various techniques such as chromatography, filtration, and precipitation to obtain highly pure antibodies.
Quality Control: The purified antibodies undergo rigorous quality control tests to ensure their specificity, potency, and absence of contaminants. These tests include assays like enzyme-linked immunosorbent assay (ELISA) and Western blotting.
Scaling up: Once the monoclonal antibodies have passed the quality control tests, the production can be scaled up to meet the desired demand. This involves optimizing the culture conditions, bioreactor parameters, and purification processes to achieve higher yields.
Challenges associated with their production
While monoclonal antibodies are important for research, diagnostics and therapeutic purposes, their production can pose several challenges. These challenges arise from various aspects of the production process and can affect the quality and scalability of monoclonal antibody production. Some key challenges include immunogenicity and tolerance, hybridoma cell line instability, optimization of cell cultures and more.
● Immunogenicity and Tolerance
Producing monoclonal antibodies (mAbs) can pose a challenge when the target antigen used for immunization is immunogenic. This means that the immunized animal may generate an immune response against the antigen, resulting in the production of antibodies that interfere with the desired mAb production or produce unwanted antibodies. As a result, it’s important to adopt various strategies to promote tolerance and reduce immunogenicity. These approaches include alternative immunization methods, antigen modifications, use of immunomodulatory agents, or the utilization of transgenic animals expressing human antibody components.
● Hybridoma Cell Line Instability
Hybridoma cell lines may occasionally lose stability over time. This instability can result in reduced antibody production, changes in antibody specificity or affinity, or loss of the desired characteristics of the monoclonal antibody.
● Optimization of Culture Conditions
It can be challenging to achieve optimal culture conditions for hybridoma cells. Various factors, such as the choice of media, growth factors, temperature and pH, can influence hybridomas’ growth and antibody production. That’s why it’s essential to carefully optimize these parameters to maximize antibody yield and maintain the desired quality of the antibodies.
● Scale-Up and Production Costs
Scaling up the production of monoclonal antibodies from small- to large-scale manufacturing can be complex and expensive. Transitioning from laboratory-scale production to commercial-scale production involves considerations such as bioreactor design, process optimization and ensuring consistent product quality. The high costs associated with large-scale production can limit the accessibility and affordability of monoclonal antibodies.
● Cell Line Development
CHO cell lines are commonly used for monoclonal antibody production due to their ability to correctly fold and glycosylate complex proteins. However, developing an optimal CHO cell line is a challenge. It involves extensive screening and selection processes to identify cells with high productivity, stability, and desirable characteristics. This includes genetic engineering techniques to enhance protein expression and stability. Furthermore, achieving consistent and reproducible production requires addressing clonal heterogeneity and ensuring the selected cell line maintains its desired characteristics over time.
● Genetic and Phenotypic Heterogeneity
CHO cell populations inherently exhibit genetic and phenotypic heterogeneity, resulting in variations in antibody expression levels, glycosylation patterns, and other critical product attributes. Managing and controlling this heterogeneity is crucial for achieving consistent and reproducible production. Techniques such as single-cell cloning and genetic engineering can help isolate and select stable and high-producing clones. Additionally, advanced analytics and process monitoring tools are employed to assess and mitigate the impact of heterogeneity on product quality and performance.
● Bioprocess Optimization
Optimizing the bioprocess parameters for CHO cell-based production systems is essential for maximizing productivity while maintaining product quality. This requires careful optimization of culture media composition, feeding strategies, temperature, pH, and dissolved oxygen levels. Balancing these factors is critical to support cell growth, maintain high antibody production rates, and ensure appropriate protein folding and glycosylation. Process optimization also involves the identification of key process parameters through design of experiments (DoE) approaches and the implementation of advanced process control strategies to maintain process robustness and stability.
Applications of monoclonal antibodies
Monoclonal antibodies are applicable in various fields, including diagnostics and therapeutics. They are highly specific and can be engineered to target specific molecules or cells, making them valuable tools in medical research and clinical practice.
Diagnostic applications
Monoclonal antibodies are extensively used in various diagnostic techniques and assays for detecting and measuring specific molecules in clinical samples. Some of the commonly used diagnostic applications of monoclonal antibodies include:
● Enzyme-Linked Immunosorbent Assay (ELISA)
ELISA is a widely employed diagnostic technique that uses monoclonal antibodies to detect and quantify specific antigens or antibodies. In a typical ELISA, a monoclonal antibody is immobilized onto a solid surface, such as a microplate, and serves as a capture antibody. The target antigen or antibody in the sample is then added to bind to the capture antibody.
The presence of the target molecule is then detected using a labeled monoclonal antibody that recognizes a different epitope on the target. The labeled antibody generates a measurable signal, such as a color change, indicating the target molecule’s presence and quantity in the sample.
● Immunohistochemistry (IHC)
IHC is a technique used for visualizing specific antigens in tissue sections. Monoclonal antibodies selectively bind to the target antigen in the tissue sample. The bound antibody is then detected using a detection system, such as a chromogen or fluorescence, to visualize the target antigen in the tissue. IHC is widely used in clinical pathology to diagnose diseases and in research settings to study protein expression and localization within tissues.
● Flow Cytometry
Flow cytometry is a powerful technique for analyzing and sorting cells based on their surface markers or intracellular components. Monoclonal antibodies conjugated to fluorochromes are used in flow cytometry to specifically bind to target molecules on the cell surface or within the cell.
The fluorescent signal emitted by the bound antibodies allows the identification and characterization of different cell populations based on their antigen expression profiles. Flow cytometry is applied in various fields, including immunology, hematology, cancer research and infectious disease diagnostics applications.
● Western Blotting
Western blotting is a technique to detect and analyze specific proteins in a complex mixture. Monoclonal antibodies bind to the target protein of interest that has been separated by gel electrophoresis and transferred onto a membrane.
The bound antibody is then detected using a secondary antibody conjugated to an enzyme or a fluorescent dye, generating a visualizable and quantifiable signal. This technique is widely used in research and clinical laboratories to study protein expression, protein-protein interactions and antibody detection.
● Rapid Diagnostic Tests
Monoclonal antibodies are integral to many rapid diagnostic tests, including lateral flow assays and immunochromatographic tests. These tests are designed for quick and point-of-care detection of specific analytes, such as infectious agents or biomarkers, in patient samples.
Therapeutic applications
Therapeutic monoclonal antibodies offer targeted and specific treatments for various diseases. They are widely used to treat cancer, autoimmune diseases, infectious diseases and other medical conditions. These therapeutic applications of monoclonal antibodies are discussed in detail below:
● Cancer Therapy
Monoclonal antibodies contribute significantly to cancer treatment. They can target specific molecules expressed in cancer cells and block the growth of tumors. Some important examples include:
Targeted Therapy: Monoclonal antibodies can be specifically designed to bind to overexpressed or dysregulated molecules in cancer cells. These antibodies can block signaling pathways that promote cancer growth or can directly induce cancer cell death. Trastuzumab for HER2-positive breast cancer and rituximab for B-cell lymphomas are some examples of targeted therapy.
Immunotherapy: Monoclonal antibodies can activate the immune system to recognize and attack cancer cells. Immune checkpoint inhibitors, including pembrolizumab and nivolumab, block molecules like PD-1 or CTLA-4, which prevent immune cells from targeting cancer cells. This helps unleash the immune response against cancer cells and has shown remarkable success in various cancers, including melanoma and lung cancer.
● Autoimmune Disease Treatment
Monoclonal antibodies modulate the immune system in autoimmune diseases where the immune system mistakenly attacks healthy tissues. These antibodies can target specific immune cells or cytokines in the autoimmune response. Some common examples include:
Anti-Inflammatory Antibodies: Monoclonal antibodies such as adalimumab and infliximab target pro-inflammatory molecules like tumor necrosis factor-alpha (TNF-α). They treat various conditions, such as rheumatoid arthritis, Crohn’s disease and psoriasis.
B-Cell Depletion: Monoclonal antibodies like rituximab specifically target and deplete B-cells, which contribute to the pathogenesis of diseases like rheumatoid arthritis and systemic lupus erythematosus.
● Infectious Disease Treatment
Monoclonal antibodies can prevent or treat infectious diseases by neutralizing pathogens or blocking their entry into host cells. Some common examples include:
Antiviral Antibodies: Monoclonal antibodies such as palivizumab and casirivimab/imdevimab prevent or treat viral infections like respiratory syncytial virus (RSV) and COVID-19.
Passive Immunization: Monoclonal antibodies can provide immediate immunity by directly neutralizing pathogens. They have been used for diseases such as rabies and botulism, requiring immediate protection.
Advantages of Monoclonal Antibodies
Monoclonal antibodies offer several advantages that contribute to their widespread use in biomedical research and clinical applications:
● Specificity
As discussed above, monoclonal antibodies can be designed to target specific molecules or cells with high precision. This specificity reduces the chances of off-target effects and allows for targeted therapy or accurate detection of biomarkers.
● Reproducibility
Monoclonal antibodies are produced through cell line propagation, ensuring consistent quality and quantity. This reproducibility is crucial for research and therapeutic purposes, as it allows for reliable results and standardized treatments.
● Low Toxicity
Monoclonal antibodies generally exhibit low toxicity compared to traditional small-molecule drugs. They are well-tolerated by the body, leading to fewer adverse effects. This makes them attractive for long-term treatments or patients with preexisting health conditions.
● Long Half-Life
Monoclonal antibodies often have a longer half-life than small molecules, meaning they can remain active in the body for an extended period. This property allows for less frequent dosing, improving patient convenience and adherence to treatment regimens.
● Versatility
Monoclonal antibodies can be engineered and modified to enhance their therapeutic properties. This includes modifying the antibody structure, such as the Fc region, to optimize antibody-dependent cellular cytotoxicity (ADCC) or complement-dependent cytotoxicity (CDC) mechanisms. It also includes conjugating them with other therapeutic or imaging agents for combination therapies or diagnostic applications.
Limitations of Monoclonal Antibodies
While monoclonal antibodies have numerous advantages, they also have some limitations that need to be considered:
● Cost
Monoclonal antibody therapies can be expensive, often making them inaccessible to some patients or healthcare systems. The complex manufacturing processes, including cell line development and purification, contribute to their high costs.
● Immunogenicity
In some cases, monoclonal antibodies can induce an immune response in patients, leading to the development of anti-drug antibodies. This immune response may reduce the efficacy of the therapy over time or cause adverse reactions.
● Limited Tissue Penetration
Being large molecules, monoclonal antibodies may face challenges in penetrating certain tissues or crossing the blood-brain barrier. This can limit their effectiveness in treating diseases that require deep tissue or central nervous system targeting.
● Development Time
The development of monoclonal antibodies p typically takes several years, from target identification to preclinical and clinical trials. This can delay access to new treatments for patients in urgent need.
● Lack of Oral Availability
Monoclonal antibodies are typically administered through injection or infusion due to their large size and susceptibility to degradation in the gastrointestinal tract. This route of administration may be less convenient for patients than oral medications.
Future direction of monoclonal antibodies
Monoclonal antibodies have contributed significantly to medicine, with ongoing research and development continuously shaping their future applications and potential. As a result, several focus areas have emerged for future directions for monoclonal antibodies.
New approaches for producing monoclonal antibodies
The traditional approach of producing monoclonal antibodies using hybridoma technology has certain limitations, including the need for animal immunization and the time-consuming process of generating stable cell lines. To reduce these limitations, researchers are exploring new approaches to improve and streamline the production of monoclonal antibodies. Some of these approaches include:
Transgenic Animals: Using transgenic animals, such as genetically modified mice, to produce human antibodies directly without humanization.
In-Vitro Antibody Production: Developing techniques for producing antibodies directly in vitro, using cell-free systems or alternative cell culture methods.
Microbial Systems: Utilizing microbial expression systems, such as bacteria or yeast, to produce antibodies more efficiently and cost-effectively.
Advances in antibody engineering and modification
Antibody engineering and modification techniques have helped develop next-generation monoclonal antibodies with enhanced properties and functions. Researchers are actively exploring ways to engineer and modify antibodies to improve their efficacy and half-life and reduce immunogenicity. Some areas of advancement include:
Antibody Fragments: Designing and utilizing smaller antibody fragments, such as single-chain variable fragments (scFv) or antigen-binding fragments (Fab), offer improved tissue penetration and manufacturing advantages.
Bispecific Antibodies: Creating antibodies that simultaneously bind to two targets enables novel therapeutic strategies and combination therapies.
Antibody-Drug Conjugates (ADCs): Conjugating monoclonal antibodies with cytotoxic drugs to deliver potent therapies directly to cancer cells increases their specificity and reduces systemic toxicity.
Fc Engineering: Modifying the Fc region of antibodies enhances their interactions with immune cells, leading to improved effector functions, such as antibody-dependent cell-mediated cytotoxicity (ADCC) or antibody-dependent cellular phagocytosis (ADCP).
Potential for combining monoclonal antibodies with other therapies
Monoclonal antibodies have demonstrated effectiveness as stand-alone therapies, but their potential can be further maximized by combining them with other treatment modalities. Researchers are exploring the possibilities of combining monoclonal antibodies with:
Immune Checkpoint Inhibitors: Combining monoclonal antibodies with immune checkpoint inhibitors, such as PD-1 or CTLA-4 inhibitors, to enhance the anti-tumor immune response.
Small Molecule Inhibitors: Using monoclonal antibodies in combination with small molecule inhibitors to target multiple pathways simultaneously, leading to synergistic effects and improved therapeutic outcomes.
Radiotherapy or Chemotherapy: Combining monoclonal antibodies with radiotherapy or chemotherapy to enhance their efficacy and overcome treatment resistance.
Gene Therapy: Using monoclonal antibodies in combination with gene therapies, such as gene editing or gene delivery systems, to improve the targeted delivery of therapeutic genes.
If you want to learn more about monoclonal antibodies, download the informational and insightful resources from Avantor. With our experts in life sciences and advanced materials, we’re contributing toward better and improved health outcomes. Visit our website, connect with our experts and be part of the Avantor community today.