An Overview of Protein Expression Systems
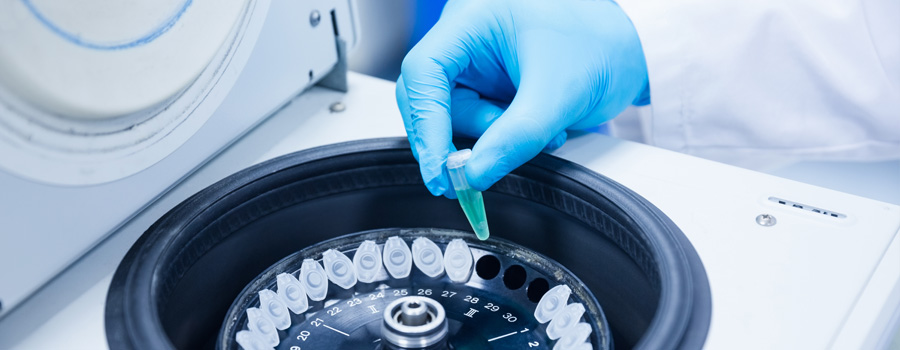
Protein expression systems play a vital role in facilitating essential biological functions. This article highlights the significance of these systems and their contribution to the expression of recombinant proteins. The four major systems for protein expression are bacterial expression systems, yeast expression systems, insect expression systems, and mammalian expression systems.
Bacterial expression systems, exemplified by E. coli, offer the advantages of speed, cost-effectiveness, and genetic manipulability. Yeast expression systems, such as S. cerevisiae, enable post-translational modifications and protein secretion. Insect expression systems, utilizing cell lines like Sf9, produce properly folded proteins with intricate modifications. Mammalian expression systems, commonly employing CHO cells, are particularly valuable for generating therapeutic proteins with complex post-translational modifications.
What are protein expression systems?
Protein expression systems refer to the mechanisms by which proteins are synthesized, modified, and regulated within living organisms. Protein expression requires the use of host organisms or cell lines to produce recombinant proteins. These host organisms can be bacteria, yeast, insect, or mammalian cells, each offering specific advantages and considerations.
In a protein expression system, a gene encoding the desired protein of interest is introduced into the host organism or cell line. This gene is typically a recombinant DNA construct created by combining the target gene with regulatory elements that control its expression. These regulatory elements ensure that the gene is transcribed into mRNA and translated into protein.
Once the gene is introduced into the host organism, it undergoes transcription, where the DNA sequence is copied into mRNA. The mRNA is then translated by ribosomes, cellular machinery that assembles the amino acids in the correct order according to the genetic code. This paves the way for ultimately synthesizing the desired protein.
Additionally, protein expression systems involve various mechanisms for modifying and regulating the synthesized proteins. Post-translational modifications, such as phosphorylation, glycosylation, and proteolytic cleavage, can occur to enhance protein stability, functionality, or localization within the cell.
The choice of a specific protein expression system depends on factors such as the complexity of the protein, the required post-translational modifications, the scale of production, and the downstream applications. Each system has its advantages and limitations regarding protein yield, quality, and the ability to perform specific modifications.
The importance of protein expression systems
Protein expression systems play a vital role in numerous areas of scientific research, medical applications, and industrial processes. These systems allow scientists to produce proteins of interest in a controlled and reproducible manner, which provides a deeper understanding of protein structure, function, and interactions.
One key importance of protein expression systems is in scientific research. By artificially synthesizing proteins, researchers can investigate their roles in biological processes, study their functions, and unravel the underlying mechanisms of diseases. Protein expression systems can allow researchers to produce proteins for biochemical and biophysical analyses. This helps facilitate the characterization of protein properties and their interactions with other molecules.
In the field of medicine, protein expression systems are critical for the production of therapeutic proteins. These systems help manufacture recombinant proteins that can be used as drugs to treat various diseases. Therapeutic proteins include antibodies, enzymes, hormones, and growth factors, which are produced in large quantities using protein expression systems (particularly mammalian expression systems) to ensure their compatibility with the human body.
Protein expression systems have significant implications in the biotechnology and industrial sectors. They are employed for the large-scale production of enzymes, industrial catalysts, biofuels, and other valuable proteins. The ability to optimize protein expression in various host organisms allows for the efficient and cost-effective production of these biotechnological products.
Furthermore, protein expression systems contribute to the emerging field of synthetic biology. By manipulating genetic elements and introducing synthetic DNA constructs, scientists can design novel proteins with desired functions or properties. Protein expression systems serve as a valuable tool in the construction and expression of these engineered proteins. This helps achieve advancements and breakthroughs in areas such as bioremediation, biosensing, and bioengineering.
Types of protein expression systems
Protein expression systems encompass prokaryotic, eukaryotic, and cell-free systems. Each type has distinct characteristics and advantages that cater to different protein production needs. Prokaryotic systems provide simplicity and high yields but may lack post-translational modification capabilities. Eukaryotic systems enable complex modifications and the production of properly folded proteins. Cell-free systems facilitate rapid protein synthesis without intact cells. The choice of a specific protein expression system depends on factors such as protein complexity, required modifications, and downstream applications.
Prokaryotic systems
Prokaryotic systems for protein expression are widely used platforms that utilize prokaryotic organisms, including Escherichia coli (E. coli). These systems hold significant importance in research, biotechnology, and industrial applications. They offer several benefits, including simplicity, rapid growth, cost-effectiveness, and high protein yields. Prokaryotic systems are particularly suitable for generating large quantities of recombinant proteins, making them valuable in high-throughput studies and industrial-scale production. However, they may not be ideal for producing proteins that require extensive post-translational modifications or proper folding, as prokaryotes lack the cellular machinery necessary for complex modifications.
Bacterial Protein Expression Systems
Bacterial protein expression systems are widely employed genetic engineering tools used to produce desired proteins within bacterial cells. These systems offer numerous benefits and advantages, making them highly popular in various fields such as research, industry, and medicine. Bacterial protein expression systems are utilized across various domains. In research, they allow for the production of recombinant proteins for structural studies, functional analyses, and protein-protein interaction studies. In the biotechnology and pharmaceutical industries, these systems are vital in producing therapeutic proteins, enzymes, and industrial enzymes for diagnostic tests and drug development .
Bacterial protein expression systems are utilized across various domains. In research, they allow for the production of recombinant proteins for structural studies, functional analyses, and protein-protein interaction studies. In the biotechnology and pharmaceutical industries, these systems are vital in producing therapeutic proteins, enzymes, and industrial enzymes for diagnostic tests and drug development .
However, it is important to note that bacterial systems may not be suitable for producing proteins that require extensive post-translational modifications or proper folding, as bacteria lack some of the cellular machinery necessary for complex modifications. In such cases, eukaryotic or cell-free expression systems may be more appropriate. Bacterial protein expression systems provide several advantages, including cost-effectiveness, scalability, and being well-studied.:
Cost-Effectiveness
Bacterial protein expression systems are highly cost-effective due to their low cultivation and maintenance expenses. Bacteria have simple growth requirements and fast replication rates, allowing for the efficient production of large quantities of recombinant proteins at a relatively low cost. This makes bacterial systems ideal for industrial-scale applications where cost-efficient production is crucial, such as in the production of enzymes, industrial catalysts, and diagnostic reagents.
Scalability
One of the key advantages of bacterial systems is their excellent scalability. Bacteria can be easily grown in large bioreactors, allowing for the efficient production of proteins on a larger scale. Their rapid growth and high cell density enable the generation of substantial protein yields. It makes them well-suited for high-throughput protein production. This scalability is particularly advantageous in industrial settings where large quantities of proteins are required for commercial applications.
Well-Studied
Bacterial protein expression systems have been extensively studied and well-characterized. They have been the focus of numerous research efforts, resulting in reliable methodologies and genetic tools that make these systems highly accessible and user-friendly. Researchers benefit from a wide range of established protocols, standardized vectors, and well-characterized host strains. This extensive body of knowledge facilitates efficient genetic manipulation and protein expression experiments, which further enables scientists to optimize protein production and streamline the research process.
Eukaryotic Systems
Eukaryotic protein expression systems offer a realm of possibilities for the production of desired proteins. By harnessing the power of eukaryotic organisms and cell lines, researchers can unlock new frontiers in protein synthesis, folding, and post-translational modifications. These systems play a vital role in fields such as biomedicine, biotechnology, and basic research, where the intricacies of protein structure and function are paramount. With their ability to generate proteins with complex modifications and high structural complexity, eukaryotic systems provide a valuable toolkit for exploring the diverse landscape of protein expression.
Yeast Protein Expression Systems
Yeast protein expression systems utilize yeast cells, particularly Saccharomyces cerevisiae, as hosts for producing recombinant proteins. A wide range of expression vectors and genetic manipulation techniques are available, which allows researchers to optimize protein expression and tailor yeast systems for specific applications.
These expression systems offer efficient protein secretion, the capability for post-translational modifications, and the ability to express complex proteins. These advantages make them valuable tools in various applications, ranging from biotechnology to the production of therapeutic proteins, where proper secretion, modifications, and complex folding are crucial for functional and biologically relevant protein production.
Efficient Protein Secretion
Yeast protein expression systems are known for their efficient secretion pathways. The well-developed secretion machinery allows recombinant proteins to be efficiently secreted into the extracellular environment. This simplifies downstream purification processes, as the proteins can be easily harvested from the culture medium. The ability to obtain high yields of pure proteins facilitates industrial-scale production and enhances the system’s cost-effectiveness, making yeast systems advantageous for large-scale protein production and downstream applications.
Post-Translational Modifications
Yeast cells possess a diverse range of post-translational modification machinery, including glycosylation. The glycosylation patterns in yeast cells closely resemble those found in human cells. It facilitates the production of recombinant proteins with proper glycosylation. This is particularly significant for therapeutic proteins, as correct glycosylation is often essential for their stability, activity, and immunogenicity. The ability to generate properly glycosylated proteins in yeast systems helps produce bioactive proteins that closely mimic their native counterparts, enhancing their efficacy and reducing potential immunogenicity issues.
Ability to Express Complex Proteins
Yeast protein expression systems can correctly fold and express complex proteins with intricate structures. The eukaryotic cellular machinery of yeast cells ensures proper post-translational modifications, protein folding, and assembly. This makes yeast systems particularly valuable for studying and producing challenging proteins, such as membrane proteins and multi-subunit complexes. The ability to express and manipulate complex proteins in yeast provides insights into their structure, function, and interactions. Moreover, the robust folding machinery of yeast cells helps produce functional and biologically relevant proteins, making yeast systems a preferred choice for various research and biotechnological applications.
Insect Cell Expression Systems
Insect cell expression systems require the use of insect cells, such as Spodoptera frugiperda (Sf9) or Trichoplusia ni (High Five), for the production of recombinant proteins. The eukaryotic nature of insect cells ensures proper protein folding, assembly, and post-translational modifications, allowing for the expression of intricate protein structures. This makes insect cell systems suitable for studying and producing proteins with complex domains, multi-subunit complexes, or proteins requiring specific modifications for their biological activity. The well-established nature of insect cell systems further contributes to their widespread use in protein expression research and development.
Efficient Post-Translational Modifications
Insect cell expression systems offer efficient post-translational modification capabilities, making them beneficial for producing recombinant proteins with complex and biologically relevant modifications. Insect cells possess a machinery that enables them to perform various post-translational modifications, including glycosylation, phosphorylation, and proteolytic processing.
One significant advantage is the ability of insect cells to add complex sugar chains through glycosylation. This process closely resembles the glycosylation patterns observed in higher eukaryotes, including humans. Proper glycosylation is vital for the functionality, stability, and immunogenicity of many therapeutic proteins. Insect cell expression systems ensure the production of recombinant proteins with native-like glycosylation patterns, enhancing their efficacy and reducing potential immunogenicity issues.
Besides glycosylation, insect cells can perform other post-translational modifications, such as phosphorylation. These modifications are key in protein regulation, cellular signaling, and protein-protein interactions.
High Protein Yields
Insect cell expression systems are renowned for their ability to achieve high protein yields, making them well-suited for industrial-scale production. The capacity of insect cells to produce large quantities of recombinant proteins is a significant advantage for applications that require substantial amounts of functional proteins.
Insect cells have robust protein synthesis machinery and can support high-level protein expression. They possess efficient transcription and translation systems. This, coupled with their ability to properly fold and process proteins, results in the generation of large quantities of correctly folded and biologically active proteins.
The high protein yield of these expression systems is particularly beneficial for applications such as vaccine production, therapeutic protein manufacturing, and the generation of diagnostic reagents. These applications often demand large amounts of functional proteins to meet clinical or commercial requirements. Insect cell systems offer a reliable and scalable platform for producing high-quality proteins, ensuring a sufficient supply for various biotechnological and pharmaceutical applications.
Mammalian Cell Expression Systems
Mammalian cell expression systems are widely regarded as the preferred platform for producing mammalian proteins due to their ability to mimic the native structure and activity of these proteins closely. These systems provide an environment that supports post-translational modifications and ensures functional activity during the production of mammalian proteins. Additionally, they offer a physiologically relevant context for studying protein function and interactions, allowing researchers to gain insights into their behavior.
Intricate Post-Translational Modifications
Mammalian cell expression systems excel at performing intricate post-translational modifications, such as glycosylation, phosphorylation, and acetylation. These systems can add complex sugar chains to proteins, resulting in glycoproteins that closely resemble those found in humans. This ensures proper folding, stability, and activity of the expressed proteins. Additionally, mammalian cells can perform other essential modifications, allowing for the generation of proteins with native-like modifications. These intricate post-translational modifications enable the production of recombinant proteins that closely resemble their natural counterparts, facilitating accurate studies of their structure, function, and interactions.
Relevant Environment for Studying Protein Function
Mammalian cell expression systems offer a physiologically relevant environment for studying protein function. By utilizing these systems, researchers can investigate the behavior and interactions of proteins in a cellular context that closely mimics the natural environment. Mammalian cells provide the appropriate cellular machinery, co-factors, and signaling pathways necessary for proper protein folding, localization, and activity. This enables the study of proteins involved in intricate cellular processes, signaling pathways, and receptor-ligand interactions with higher accuracy and relevance. The physiological relevance of mammalian cell expression systems enhances our understanding of protein function in the context of human biology.
High Levels of Protein Expression and Secretion
Mammalian cell expression systems are known for achieving high levels of protein expression and secretion. These systems possess robust protein synthesis machinery, efficient transcription and translation systems, and proper protein folding mechanisms. As a result, they can produce large quantities of correctly folded and biologically active proteins. The high protein expression and secretion capabilities of mammalian cell expression systems are particularly advantageous for applications that require significant amounts of functional proteins, such as therapeutic protein production and research studies requiring substantial protein yields.
Cell-Free Systems
Cell-free systems offer a unique approach to protein expression by allowing rapid protein synthesis without the need for intact cells. In these systems, the protein synthesis machinery is extracted from cells and used in a controlled environment. This enables efficient production of proteins with high yields and provides researchers with more flexibility in terms of experimental design and protein engineering. These systems are particularly beneficial for applications that require quick protein synthesis, protein labeling, or the production of toxic proteins that might be harmful to living cells. Their versatility and speed make them valuable tools in various research fields, including synthetic biology, protein engineering, and drug discovery.
In-Vitro Protein Synthesis
In-vitro protein synthesis is a type of cell-free system that involves the direct synthesis of proteins using purified components in a test tube or reaction mixture. This system offers several benefits, including rapid protein production, high yields, and simplified purification processes. However, it also has various disadvantages including lack of cellular environment, co-factor limitations, complex protein challenges, and scale limitations.
This type of synthesis is applicable in various research areas, such as high-throughput protein screening, structural biology, and protein engineering. Its flexibility, speed, and ease of use make it a valuable tool for producing small quantities of proteins for research purposes or for the production of customized proteins with specific modifications.
Advantages
- Rapid Protein Production
In vitro systems offer high protein yields by optimizing reaction conditions and eliminating competing cellular processes. Larger quantities of the desired protein can be obtained with efficient protein synthesis and accumulation. This is beneficial for applications that require substantial amounts of protein, including biochemical assays, structural studies, and therapeutic protein production.
- Simplified Purification
In-vitro protein synthesis eliminates the need for cellular membranes and organelles, simplifying the purification process. The absence of cellular debris reduces the complexity of downstream purification steps, resulting in higher purity and yield of the target protein. This simplification saves time and effort in the purification workflow, making in vitro systems more streamlined and efficient.
- Flexibility and Control
In-vitro systems offer the desired control over reaction conditions, substrate concentrations, and labeling options. Researchers can fine-tune protein expression levels and incorporate specific modifications or isotopic labels tailored to their research needs. This flexibility allows for customized protein production and facilitates studies in structural biology, protein engineering, and functional characterization, where precise control over protein parameters is crucial for obtaining accurate results.
Disadvantages
- Lack of Cellular Environment
In-vitro protein synthesis lacks the intricate cellular machinery required for proper protein folding and post-translational modifications. Without the assistance of chaperones and other cellular components, some proteins may fail to fold correctly or miss out on crucial modifications, leading to compromised functionality or reduced activity. This limitation can particularly impact proteins that rely on complex folding pathways or intricate structural rearrangements to achieve their native conformation and function.
- Co-Factor Limitations
Certain proteins depend on co-factors or chaperones that are absent in the cell-free system. Without these essential components, the expression or proper functioning of specific proteins can be hindered. Co-factors play crucial roles in enzymatic reactions, protein stabilization, or protein-protein interactions. The absence of these co-factors in in-vitro systems can limit the functionality and activity of such proteins, which further restricts their applications in certain research areas.
- Complex Protein Challenges
In-vitro systems may encounter complications when expressing complex proteins with intricate structures or multi-subunit assemblies. The correct folding and assembly of these proteins often require the coordinated action of various cellular components, which are absent in the cell-free environment. Achieving the proper folding and assembly of such complex proteins without the cellular environment can be challenging, resulting in reduced yields, misfolded conformations, or functional impairments.
- Scale Limitations
In-vitro protein synthesis systems are typically more suitable for small-scale production rather than large-scale industrial applications. Scaling up the system for mass production of proteins may present challenges, including increased costs and technical limitations. The absence of cellular growth and replication mechanisms in in-vitro systems limits the scalability and cost-effectiveness compared to other expression systems, such as bacterial or mammalian cell-based systems, which can be more readily adapted for large-scale protein production.
Advantages and Disadvantages of Protein Expression Systems
Protein expression systems offer numerous advantages, making them valuable tools in biological research, biotechnology, and pharmaceutical industries. These systems allow for the production of recombinant proteins with diverse applications, contributing to advancements in medicine, agriculture, and basic science. Understanding these advantages and disadvantages is crucial in selecting the most suitable protein expression system for specific research or production goals, considering factors such as protein complexity, desired modifications, scalability requirements, and cost-effectiveness.
Advantages
- Versatility
Protein expression systems provide a versatile platform for producing various proteins with diverse functions and applications. Researchers can produce enzymes for industrial processes, antibodies for diagnostics and therapeutics, hormones for medical treatments, and structural proteins for studying cellular components. This versatility allows for in-depth investigations into protein function, disease mechanisms, and the development of novel therapeutic interventions.
- Customization
Expression systems offer the flexibility to customize protein production by incorporating specific modifications. Researchers can add tags or fusion partners to facilitate protein purification, characterization, and tracking. Isotopic labels can be introduced for structural and functional studies using techniques such as nuclear magnetic resonance (NMR) or mass spectrometry. This customization enables researchers to tailor protein expression to their specific research needs, enhancing the understanding of protein properties and interactions.
- High Yields
Optimized protein expression systems can achieve high yields of recombinant proteins. This is particularly advantageous for applications requiring large quantities of proteins, such as industrial enzyme production, vaccine manufacturing, or structural biology studies. High protein yields improve efficiency, reduce costs, and ensure an adequate supply of proteins for downstream applications, allowing for more extensive experimentation and broader applications.
- Post-Translational Modifications
Eukaryotic expression systems, including yeast and mammalian cells, can help perform complex post-translational modifications. These modifications, such as glycosylation or phosphorylation, play crucial roles in protein structure, stability, activity, and immunogenicity. Eukaryotic systems closely mimic the native cellular environment, allowing for the production of biologically relevant proteins with proper modifications. This is particularly important for therapeutic proteins, as correct post-translational modifications can enhance efficacy and reduce immunogenicity.
Disadvantages
- Cost and Complexity
Protein expression systems, particularly those involving mammalian cells, can be costly and complex to establish and maintain. The requirement for specialized culture media, sterile environments, and stringent quality control measures increases the overall expenses and complexity of the system. The need for skilled personnel and sophisticated laboratory infrastructure further adds to the costs, making these systems less accessible for some research groups or smaller-scale applications.
- Time-Consuming Optimization
Achieving optimal protein expression levels and maintaining protein quality often entails extensive optimization of various parameters. Researchers must fine-tune expression vectors, select suitable host cells, optimize induction conditions, and culture parameters. This optimization process can be time-consuming and resource-intensive, requiring multiple iterations and experiments to achieve desired protein yields and functionality. The need for optimization can delay research timelines and increase overall project duration.
- Protein Misfolding and Aggregation
Heterologous expression systems may encounter challenges related to protein misfolding and aggregation. The absence of specific cellular factors or differences in the cellular environment between the expression system and the native host can result in improper folding and the formation of protein aggregates. This can lead to reduced protein functionality and lower yields. Overcoming protein misfolding and aggregation issues often requires additional strategies, such as co-expression of chaperones or implementing specific folding conditions, which further adds complexity to the expression process.
- Limited Scalability
Not all protein expression systems are easily scalable for large-scale production. While bacterial systems, for example, are often scalable and cost-effective, other systems like mammalian cells may face challenges in scaling up to meet industrial demands. Factors such as growth requirements, production stability, and downstream purification processes can limit scalability. Developing robust large-scale production strategies for certain expression systems may require additional investments in equipment, process optimization, and production facility infrastructure.
What Techniques are Used for Protein Expression?
Protein expression techniques are employed to produce recombinant proteins in laboratory settings. Traditionally, these techniques involved introducing a DNA vector containing the gene of interest into host cells through transfection or transformation. Inside the cells, the genetic information is transcribed into messenger RNA (mRNA) and subsequently translated into protein by the cellular machinery. These techniques encompass a range of approaches and technologies, each with its own advantages and considerations. The selection of the most suitable technique depends on various factors, including the specific protein of interest, the choice of host cells, the required protein yield, downstream applications, and experimental timelines. Researchers carefully consider these factors to determine the optimal protein expression technique for their specific goals and requirements.
Transient Transfection
Transient transfection involves the introduction of a DNA vector into host cells, leading to the production of the desired protein over a short period. It is useful for obtaining a quick supply of recombinant protein but is not suitable for long-term protein production. The transient nature of gene expression makes it ideal for experimental purposes, such as protein characterization or functional studies.
Stable Transfection
Stable transfection involves the integration of the DNA vector into the host cell's genome, allowing for continuous production of the desired protein. This technique is suitable for long-term protein expression and the generation of stable cell lines. Stable transfection enables consistent protein production, making it valuable for large-scale protein production or applications that require a constant supply of the protein.
Inducible Expression Systems
Inducible expression systems provide control over protein expression by using inducible promoters. The expression of the target gene can be regulated by adding specific inducers, such as chemicals or temperature shifts. This enables precise control over protein production, allowing researchers to fine-tune expression levels and optimize protein yields. Inducible expression systems are particularly useful when tight regulation of protein expression is required or when studying proteins with toxic or detrimental effects on host cells.
Cell-Free Expression Systems
Cell-free expression systems involve protein synthesis outside of intact cells, typically using cell lysates or extracts. These systems offer advantages such as rapid protein production, flexibility, and the ability to incorporate specific modifications. By bypassing the need for living cells, cell-free systems provide a simplified and controlled environment for protein synthesis. They are useful for applications requiring fast protein production, custom modifications, or the study of toxic proteins.
In-Silico Prediction and Design
In-silico prediction and design involve the use of computational tools and algorithms to predict protein expression levels, optimize codon usage, and enhance protein solubility. These techniques aid in the design and engineering of protein expression systems to improve protein production and functionality. By analyzing protein sequence characteristics and codon usage patterns, researchers can optimize the expression system parameters and predict the yield and behavior of the recombinant protein. In silico prediction and design contribute to the efficient design of protein expression experiments and the successful production of desired proteins.
How to Select a Protein Expression System
When selecting a protein expression system, several factors need to be considered to ensure the optimal production of the protein of interest. This helps researchers make an informed decision when selecting the optimal protein expression system that aligns with the specific requirements of their protein of interest. By matching the characteristics of the protein with the capabilities of the expression system, successful production of functional and high-quality recombinant proteins can be achieved.
Structural Complexity
Proteins with complex structures or intricate domains often require eukaryotic expression systems like yeast or mammalian cells. These systems provide the necessary cellular machinery and environment for proper folding and functionality, ensuring that the protein attains its native conformation and activity.
Post-Translational Modifications
Eukaryotic systems, such as yeast or mammalian cells, are preferred when post-translational modifications like glycosylation, phosphorylation, or proteolytic processing are crucial for protein function. These systems possess the machinery to perform these modifications accurately, ensuring the production of biologically relevant and fully functional proteins.
Solubility
Protein solubility is a key consideration in expression system selection. For proteins prone to aggregation or insoluble inclusion body formation, bacterial systems like Escherichia coli are commonly employed. Strategies like co-expression with chaperones or fusion tags can assist in enhancing solubility. Eukaryotic systems , on the other hand, may offer better solubility due to their more elaborate folding and quality control mechanisms.
Cellular Localization
Understanding the cellular localization requirements of the protein is essential. Proteins needing specific subcellular compartments, such as membrane proteins, benefit from expression in insect or mammalian cells. These systems ensure correct membrane insertion, post-translational modifications, and provide the appropriate environment for protein function.
Investment
The financial and resource investments associated with expression systems should be considered. Bacterial systems, such as E. coli, are cost-effective and easier to handle, making them suitable for routine laboratory use. On the other hand, eukaryotic systems, including yeast or mammalian cells, may require higher investments due to specialized facilities, more complex culture conditions, and increased reagent costs. Evaluating the available resources, expertise, and specific experimental goals is important when determining the most suitable expression system.
Protein express products and related resources are available here. With a strong commitment to scientific excellence, Avantor offers a diverse range of innovative laboratory products, including reagents, consumables, equipment, and analytical instruments. Shop now and discover how Avantor can support you in advancing your scientific discoveries.